The term bioavailability is, in pharmacology, explained as “a subcategory of absorption and is the fraction (%) of an administered drug that reaches the systemic circulation.”
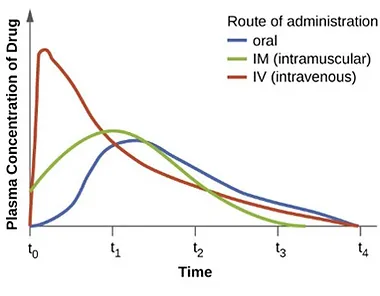
When the medication is administered intravenously (directly into a person’s vein), its bioavailability is 100 %. However, when a medication is administered via routes other than intravenous, its bioavailability is generally lower (Figure 1) due to intestinal endothelium absorption and first-pass metabolism [2]. The medication administered orally is absorbed by the digestive system – the intestinal barrier prevents the passage of gut microbiota into the circulation, yet allows nutrients or drugs to be absorbed. The absorbed active drug is then carried through the portal vein into the liver, where most of it is metabolized. The first pass through the liver generally greatly reduces the bioavailability, as only a small amount of active drug finally enters the circulatory system [2]. However, oral administration of the drug is much more patient-friendly and can be safely used at home.
Nanoparticulate drug delivery systems have shown important advantages in stability and bioavailability, such as:
- protection of the drug from premature degradation and interaction with the physiological environment
- increased intracellular penetration
- increased solubility of insoluble drugs
- enhanced drug absorption [3]
Smaller particles have larger surface area, which improves bioavailability
The research has shown that smaller particles, preferably of 100 nm [4], significantly improve the bioavailability of the drug. The drug-containing particles have to pass the physiological barriers, such as mesh-like mucus barrier that is present on the top of intestinal epithelium. The uppermost layer consists of loose mucus that can be easily removed by suction and shear; however, the firm mucus underneath adheres firmly to the epithelium surface and is resistant to the removal. It was reported that particles of 40 nm and 100 nm reach into deep, folded surfaces of the colorectal epithelium, whereas bigger particles, 200 nm and 500 nm, just spread over the surface of the epithelium [5].
Nanoparticles enter the cells via endocytosis
Small and non-polar molecules permeate cell membranes via cell diffusion but most nanoparticles are polar molecules and enter cells via endocytosis. First, nanoparticles need to adhere to the cells and it was reported that larger surface area is likely to lead to a stronger adhesion to the cell. The adhered particle can then be internalized by a living cell. There are four types of endocytic pathways, namely phagocytosis, macropinocytosis, clathrin-mediated endocytosis and caveolin-mediated endocytosis [3].
The internalization pathway depends on the characteristics of the nanoparticle as it also defines in which part of the cell or organelle the nanoparticle will end up. It was reported that smaller solid lipid nanoparticles (SLNs) showed improved bioadhesion in the gastrointestinal tract, which prolonged the residence time and extended period of time for drug release at the target site [3]. The smaller nanostructured lipid carriers (NLC – 100 nm) showed higher uptake efficiency in Caco-2 cell, as well as higher penetration ability in Caco-2 cell monolayer, compared with NLC-200 nm and NLC-300 nm [4]. Zhao et al. reported that the 100 nm nanovaccine exhibited better pharmacokinetic efficacy than the 500 nm nanovaccine in the presence of alum adjuvant [6]. Decreasing the size of retinoic acid-SLNs, from 328.8 nm to 89.3 nm, improved the bioavailability 3-fold [7]. These are just a few examples that show how particle size affects the bioavailability of the orally administered drug.
Surface properties and particles size play an important role
Moreover, the surface properties and particle morphology of the drug delivery vehicle play an important role for oral bioavailability as well. Surface chemistry of nanoparticles can greatly impact their interactions with biological pathways and alter efficacy [3]. For example, PEGylated nanocarriers have increased mucus penetration. PEG attachment also reduces recognition and clearance and promotes drug accumulation, whereas coating nanoparticle with tannic acid (TA) improves solubility of hydrophobic molecules and such particles avoid gastric acid degradation [3].
Designing an efficient drug delivery system requires many different aspects to be considered for each drug individually, including particle size, surface modifications, morphology and formulation composition [6].
Dyhydromatics ShearJet® Processors
Our Dyhydromatics Reaction Chamber® modules and ShearJet® processors are well-suited for fabrication of small spherical nanoparticles for drug delivery. Our hydraulic lab scale ShearJet® HL60 with low dead volume was made for R&D purposes. Due to the Reaction Chamber® technology, the established process is linearly scalable to Dyhydromatics pilot or production scale high-shear processors. If you are interested in our equipment, a proof-of concept testing of your application or you want to learn more about our technology, do not hesitate to reach out to our friendly customer service. We look forward to answer your questions. Visit our website at www.dyhydromatics.com.
Literature
[1] Choudhary, Ankur. drug-bioavailability.jpeg. [Created date: unknown], [Access date: 30th June 2023]. Website: https://www.pharmaguideline.com/2021/07/bioavailability-of-drugs.html.
[2] Herman TF, Santos C. First Pass Effect. 2022 Sep 24. In: StatPearls [Internet]. Treasure Island (FL): StatPearls Publishing; 2023 Jan–. PMID: 31869143.
[3] Wang, Y., Pi, C., Feng, X., Hou, Y., Zhao, L. & Wei, Y.: The Influence of Nanoparticle Properties on Oral Bioavailability of Drugs. Int J Nanomedicine, 2020;15:6295-6310, DOI: 10.2147/IJN.S257269
[4] Li, H., Chen, M., Su, Z., Sun, M., Ping, Q.: Size-exclusive effect of nanostructured lipid carriers on oral drug delivery. Int J Pharm 2016;511(1):524-537. doi: 10.1016/j.ijpharm.2016.07.049
[5] Maisel, K., Ensign, L., Reddy, M., Cone, R., Hanes, J.: Effect of surface chemistry on nanoparticle interaction with gastrointestinal mucus and distribution in the gastrointestinal tract following oral and rectal administration in the mouse. JCR, 2015;197:48-57. doi: 10.1016/j.jconrel.2014.10.026
[6] Zhao Z, Hu Y, Hoerle R, et al. A nanoparticle-based nicotine vaccine and the influence of particle size on its immunogenicity and efficacy. Nanomedicine. 2017;13(2):443–454. doi: 10.1016/j. nano.2016.07.01564
[7] Hu, LD., Tang, X., Cui, FD.: Solid lipid nanoparticles (SLNs) to improve oral bioavailability of poorly soluble drugs. J. Pharm. Pharmacol. 2004;56(12):1527–1535. doi: 10.1211/0022357044959
[8] Zhuang, CY., Li, N., Wang, M., Zhang, XN., Pan, WS., Peng, JJ., Pan, YS., Tang, X.: Preparation and characterization of vinpocetine loaded nanostructured lipid carriers (NLC) for improved oral bioavailability. Int J Pharm, 2010;394(1-2):179-185, doi: 10.1016/j.ijpharm.2010.05.005